Measuring the True Costs and Benefits of Energy Storage
Part Two - How cycle life and environmental factors impact LCOE
In last month’s issue, Part One of this series began covering how checking a few simple performance metrics can help calculate the true costs and benefits of competing energy storage systems. Additionally calculating this levelized cost of energy (LCOE) can help distinguish between what might look like a good price up front from what’s actually a better buy in the long run.
How to Calculate the Levelized Cost of Energy (LCOE)
To calculate the Cost of Electricity in Kilowatt Hours (kWh) over time:
Step One: Gather the Facts
- Size of Battery in Rated Amp Hours – Ah
- Voltage of Battery – V
- Depth of Discharge – %
- Number of Batteries – Qty
The battery rating is based on the manufacturer’s stated capacity in watt hours at specific discharge rates.
Step Two: Calculate Watt Hours (Wh)
- Wh = Qty x Ah x V x %
Step Three: Calculate Lifetime Watt Hours (LW)
- LW = Wh x Cycle Life
Cycle Life is the number of full (not partial) charge and discharge cycles expected over a battery’s lifetime while it has at least 80% of its original published capacity. It is based on manufacturer's estimate using specific depth and rates of discharge and operating temperatures.
Step Four: Factor in Costs
- Price (per battery)
- Calculate Total Battery Cost (Qty x Price)
- Add Ancillary Costs including Cabling, Racking, Containment, Venting, Cooling, Installation, Transportation, Maintenance, etc.
- Calculate Actual System Cost = Total Battery Cost + Ancillary Costs
Step Five: Calculate Cost per Wh
- Cost per Wh = System Cost / LW
Step Six: Calculate LCOE in kWh
- LCOE = LW x 1000
The various components of the LCOE equation were covered in last month’s issue. These include, but are not limited to, whether a battery system needs HVAC equipment and extra space to regulate temperature regulation, maintenance and the manpower required to conduct it, use of other chemicals or resources, limitations on usages, operation locations or transport methods because of temperature issues, or thermal runaway risks posed by using cobalt oxide.
Battery chemistries containing cobalt include Lithium Cobalt Oxide (LiCoO2 or LCO), Lithium Nickel Manganese Cobalt Oxide (LiNiMnCoO2 or NMC), and Lithium Nickel Cobalt Aluminum Oxide (LiNiCoAlO2 or NCA). This grouping of LI batteries can be referred to as LI with cobalt. Chemistries without cobalt oxide include Lithium Ferrous Phosphate (LiFePO4 or LFP), and Lithium Titanate (Li4Ti5O12 or LTO), although LTO is rarely used in large format energy storage. For more on this topic, please see Part One in the January/February issue.
Cycle Life
The LFP chemistry offers a significantly longer cycle life, meaning the number of times the battery can be fully charged and discharged, than LI with cobalt. It also allows a deeper discharge depth and a faster charge/discharge rate.
Based on published studies, utilizing an 80 percent depth of discharge (DOD), Li batteries with cobalt offer a cycle life of 500 to 700 cycles, whereas the LFP batteries offer 2,500 to 4,000+ cycles. Therefore, if an Li battery with cobalt is discharged by 80 percent, once a day for a year, it would offer a cycle life of about two years (700/365 = 1.9 years). If the individual battery is discharged at a shallower depth, it could last longer. But that would result in less useable kWh per pound, square foot, and human or mechanical resources required to install, house, and maintain the system. A shallower depth of discharge per battery (to extend cycle life) would also require more batteries, installation costs, space, and maintenance to provide the same amount of power; raising the cost of energy over time.
By contrast, an LFP battery that is discharged by 80 percent once a day for a year will provide the same amount of power for 6.8 years to 10.9 years (2,500/365 = 6.8 and 4,000/365 = 10.9). That’s more than three to five times as long. Therefore, the true cost of LFP battery storage over time is far lower given its longer cycle life, compact footprint and more efficient utilization of resources, even though its up-front price-point might be higher than batteries with other chemistries such as Li with cobalt, lead acid, Lithium Nickel Manganese Cobalt, flow, zinc bromide, or salt-water-based batteries.
Additionally, independent tests reported in scientific papers (Journal of Power Sources Vol. 257, 2014) studying the cycle life of LFP battery cell chemistry demonstrate cycle life in excess of 8,000 cycles is practical and can be maintained when the depth of discharge is limited to a range of 60 percent. Therefore, if LFP batteries are integrated into a small or large power storage format in which the monitoring and control software platform maintains a recommended depth of discharge, the batteries could provide as many as 21 years of useful life (8,000/365 days = 21.9 years). This extended cycle life for the LFP chemistry can also be enhanced by a conservative rate of charge and discharge.
One other point to consider is that so-called “long duration, large format” batteries, such as aqueous salt water and some flow batteries, require an unusually slow optimal discharge rate. These battery chemistries can require discharge rates, as long as up to 20 hours, to protect capacity (useable kWhs) and cycle life. This significantly adds to the size, weight, and LCOE per kWh. Meanwhile, the rate of charge and discharge for Li with cobalt batteries must be heavily regulated to control the buildup of heat and to prevent thermal runaway.
When considering an energy storage solution, be sure to calculate the LCOE and any other impact in order to assess the true costs and benefits of the system.
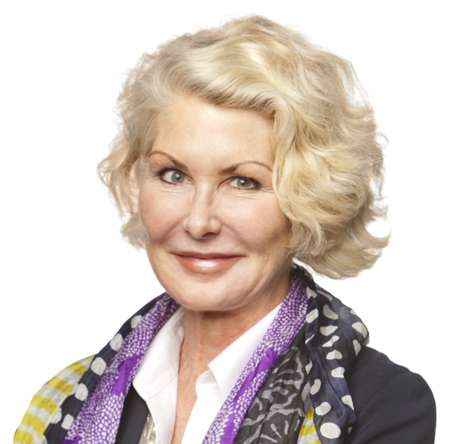
SimpliPhi Power | www.simpliphipower.com
Volume: 2016 March/April